January 13, 2025 5g, Graphene, Scientific alternative studies
Graphene’s magnetic stability and ability to magnetically change spin properties is clear.
Graphene Spintronics. Has Pandora’s Box been opened ?
Reference study
Maassen, J.; Ji, W.; Guo, H. (2011). Graphene spintronics : the role of ferromagnetic electrodes. Nano letters, 11 (1), pp. 151-155. https://doi.org/10.1021/nl1031919
Spintronics Introduction
Spintronics is the branch of science and technology concerned with the study of the charge and spin of electrons.
A “spin” is the rotation of the electron around itself.
It takes on a unique value (quantum number) that is equivalent to the orbital angular momentum.
Its applications in quantum computing take advantage of the ability to reflect qubits or quantum bits (Burkard, G.; Engel, HA; Loss, D. 2000 | Leuenberger, Minnesota; Loss, D. 2001), similar to what a computer would do, by changing their spin values using magnetic nanofields.
This is possible because of the magnetoresistance effect of electrons and their spins, and spin valves with high sensitivity to magnetic fields have been developed (Gergs, NM; Bender, SA; Duine, RA; Schuricht, D. 2018 | Braun, M.; König, J.; Martinek, J. 2006), which demonstrate the feasibility of the concept.
Analyzed facts
The study analyzes how to make graphene spintronics work using ferromagnetic cobalt-nickel electrodes, which achieve spin-spin efficiencies on the order of 60-80%, meaning good computational interaction capability.
In other words, it is possible to transfer the principles of binary and quantum computing, as in a processor or a PC chip on a motherboard, to graphene at the nanoscale.
It is stated that “this large spin filtering (related to the incidence of the induced magnetic field) results from the material-specific interaction between graphene and FM (ferromagnetic interface), which destroys the linear dispersion relation of the graphene bands, which in turn modifies the linear dispersion relation of the graphene bands and leads to the opening of spin-dependent energy gaps“.
This is the ability to change the physical properties of spin at will, to alter its natural values.
“The minority spin forbidden band is at a higher energy than the majority spin forbidden band…, a property that leads to large currents dominated by minority spins“.
In other words, by changing the spin energy current, it is possible to change both its angular momentum and its polarization, and thus detect a different value at will.
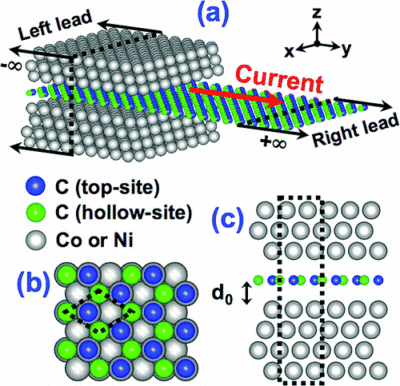
The authors introduce the article by stating the goal of spintronics and magnetoelectronics as follows : “The field of spintronics or magnetoelectronics uses the degree of freedom of electron rotation and its intrinsic magnetic moment to influence or control the properties of a circuit“.
Clearly, researchers are working to develop circuits, transistors, and eventually nanoscale chips or processors.
In this sense, graphene has all the properties necessary for the development of spintronic devices, as reported below : “Graphene, a 2D (two-dimensional) lattice of C (carbon) atoms, is a gapless material with linearly scattering electronic bands that bind at the Fermi level in conical (Dirac) points located at K-points in the Brillouin region.
Graphene has received much attention for its exceptional properties, including effective zero-mass carriers with extremely high mobilities, and is poised to play a role in the future of nanotechnology.
Among other properties, graphene has a weak spin-orbit interaction due to the low atomic number of C (carbon), resulting in long spin coherence lengths.
Therefore, graphene is a promising material for applications in spintronic devices”.
In this statement, the magnetic stability of graphene and its ability to magnetically change its spin properties is clearly evident.
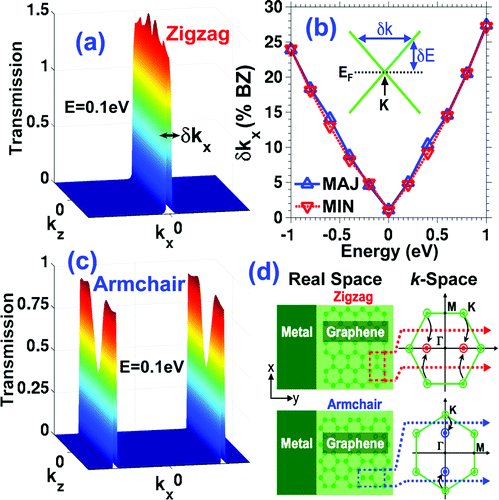
However, the authors note some problems they are trying to solve in the development of their work : “The generation and injection of a spin-polarized current in graphene is crucial for the development of graphene-based spintronics.
In theory, nanolayers are expected for graphene, unlike pure graphene, which has a local magnetic moment at zigzag edges, but a significant limitation arises in the presence of edge clutter and impurities, which have been shown to suppress this magnetic state.
Therefore, efficient spin injection into graphene is necessary for the fabrication of a prototypical spintronic device“.
Clearly, the researchers are running into the problem of impurities in the material.
It should not be forgotten that the paper was published in 2011.
Since then, techniques and methods have been developed to achieve graphene of the highest purity and quality, a topic that has been widely discussed in the scientific literature (Konwar, S.; Dhapola, P.S.; Gupta, M.; Singh, R.C.; Singh, P.K. 2019 | Bu, Y.; Liang, H.; Gao, K. Zhang, X.; Shen, X.; Zhang, J. 2020 | Manoratne, C.H.; Rosa, S.R.D.; Kottegoda, I.R.M. 2017 | Rosillo-Lopez, M.; Salzmann, C.G. 2016 | Jasim, D.A.; Lozano, N.; Kostarelos, K. 2016 | Zhao, M.Q.; Zhang, Q.; Huang, J.Q.; Tian, G.L.; Chen, T.C.; Qian, W.Z.; Wei, F. 2013).
Of course, in the years before this “plandemic” this problem had already been solved, so the degree of precision for spintronic processing will increase very much.
In fact, the book on graphene spintronics by (Jozsa, C. van-Wees, BJ 2019) deals with “spin dynamics in graphene field effect transistors with ferromagnetic injector and detector contacts; effects related to spin transport in graphene; the Fermi level for modifying magnetism and spin spin by electrostatic gating to load graphene with electrons or gaps at a density to create a field effect transistor type device; the method of detecting spin transport electrically through a two-terminal spin valve where graphene is contacted by two ferromagnetic electrodes“.
To build the spintronic device for the experiment, a 2D graphene sheet was placed between two ferromagnetic cobalt-nickel electrodes, “in which the spin-polarized current is mainly dominated by the coupling between the layers. …. In addition, the in-plane current geometry, where transport occurs parallel to the graphene, is the most common experimental and theoretical device architecture.
In such systems, the source and drain electrodes consist of FM-coated graphene (ferromagnetic materials), which, depending on the nature of the chemical bonds, can hybridize and result in a complex electronic structure“.
In the case of the research, cobalt-nickel hybridizes strongly with graphene.
Despite this, “it is critical to properly characterize the detailed atomic structure in order to obtain the correct electronic states at the interface and accurately analyze the polarized spin transport properties of the device.
Given the nature of this problem, it is necessary to use an ab-initio atomistic model for a precise treatment of the contact chemical interaction“.
This statement proves beyond doubt the possibility of operating nanotransistors on a near-atomic scale in the form of graphene by modifying magnetic fields and thus electromagnetic wave emissions, given the absorption properties of graphene ( Avdoshenko, SM; Ioffe, IN; Cuniberti, G . Dunsch, L .; Popov, AA 2011 | Ray, SC; Soin, N.; Makgato, T.; Chuang, CH; Pong, WF; Roy, SS; McLaughlin, JA 2014 | Hashmi, A.; Hong, J. 2014 | Wang, J.; Xu, X.; Mu, X.; Ma, F.; Sun, M. 2017).
On the other hand, it is worth noting that ferromagnetic electrodes can also be made of Fe3O4 or magnetite, a material that usually integrates graphene oxide, as can be seen from the following magnetoresistance studies for spintronics, see (Liao, ZM; Wu, HC; Wang , JJ; Cross, GL; Kumar, S . Shvets, IV; Duesberg, GS 2011 | Kharissova, OV; García, BO; Kharisov, BI; Méndez, UO 2016 | Tsuchiya, T .; Terabe, K.; Ochi, M.; Higuchi, T.; Osada, M.; Yamashita, Y.; Aono, M. 2016).
Other studies
In the work of (Soriano, D.; Munoz-Rojas, F.; Fernández-Rossier, J.; Palacios, JJ 2010) we explore “how hydrogenation of graphene nanonayers at low concentrations can open spaces towards carbon-based spintronics applications, independent of any specific edge termination or passivation of the nanonayers“.
This may suggest that hydrogen can interact with graphene to configure the electrodes needed to make the transistor, further simplifying the transistor model.
In fact, this is confirmed in the following statement : “Density functional theory calculations show that an absorbed H (hydrogen) atom induces a spin density near orbitals whose symmetry and degree of localization depend on the distance from the edges of the nanolayer.
Later they add: “Under the influence of a magnetic field, hydrogenated AGNR (graphene nanotape) behaves as a dilute paramagnetic semiconductor for small concentrations of H (hydrogen).
At large concentrations, where the spin density is zero everywhere, the influence of the field can only lead to a small diamagnetic response.
At intermediate concentrations, where the magnetization clouds induced by the H (hydrogen) atoms interact with each other, it is possible to go from the AF state to the F state by applying a sufficiently strong magnetic field”.
This shows that applying magnetic fields to graphene transistors affects their wireless programming and, most likely, their functional/operational performance.
On the other hand, hydrogen electrodes can induce the magnetization of graphene, which would explain the electromagnetic properties mentioned in the article (González-Herrero, H .; Gómez-Rodríguez, JM; Mallet, P .; Moaied, M .; Palacios, JJ; Salgado, C .; Brihuega, I. 2016).
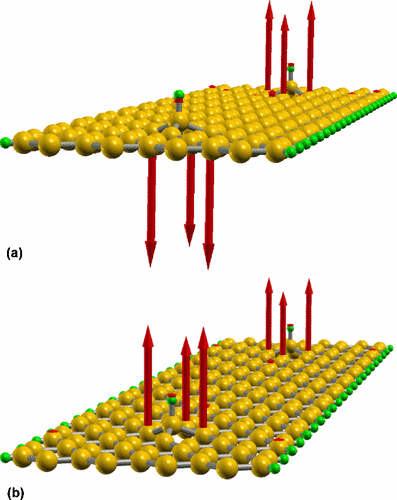
In addition, the research by (Li, L.; Qin, R.; Li, H.; Yu, L.; Liu, Q.; Luo, G.; Lu, J. 2011) analyzes “the possibility of functionalized graphene as a high-performance two-dimensional spintronic device.
It was found that graphene functionalized with O (oxygen) on one side and H (hydrogen) on the other side in the electrode conformation forms a ferromagnetic metal with spin filter efficiency up to 54% with finite polarization… The spin valve is controlled by applying a magnetic field to stabilize its ferromagnetic state.
The resulting room temperature magnetoresistance is up to 2200%, which is an order of magnitude higher than available experimental values.”
In the end, they conclude that “compared to ultrathin graphene nanolayer spintronic devices, functionalized graphenes allow a much higher current with fewer fabrication requirements and are more competitive“.
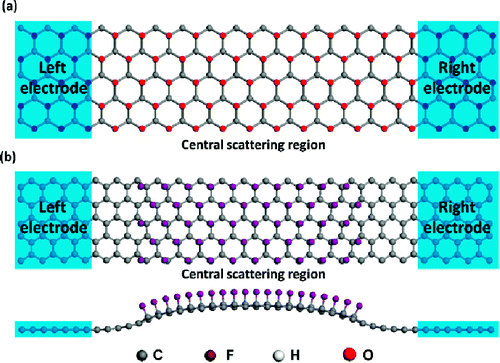
Another property that has been investigated is the switching of spin rotation in quantum dots.
According to (Gergs, NM; Bender, SA; Duine, RA; Schuricht, D. 2018), it is possible to switch the spin rotation to change its properties and values to encode the necessary computational values.
This is reflected in their following statement : “We demonstrate that quantum transport through a spin-degenerated quantum dot provides unique control over the spin pairs acting on the attached nanomagnets, allowing the effective switching of nanomagnets from a parallel to an antiparallel configuration and vice versa“.
This shows that it is possible to develop nanoelectronics or spintronics based on all types of semiconductors, including graphene and its derivatives.
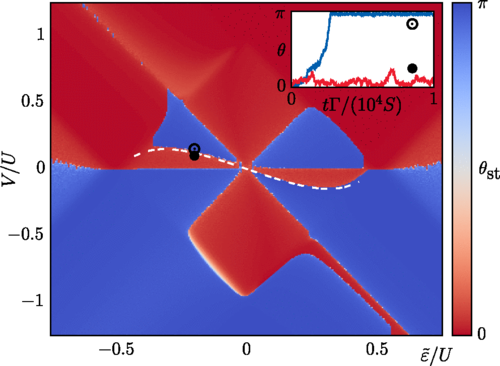
The study by (Akram, KB; ul-Hassan, SM; Ahmed, A .; Hamayun, MA; Rafique, M .; Manzoor, S. 2020) is characterized by the special property of reduced graphene oxide rGO/Fe3O4 to exhibit “giant” AC (alternating current) magnetoconductivity, which is essential for semiconductor transistor assembly.
Specifically, they state that “the structural, AC transport and magnetotransport properties of reduced graphene oxide (rGO) composites and magnetite nanoparticles were measured in the frequency range from 10 Hz to 2 MHz and in static magnetic fields up to 500 mT“.
In conclusion, they state that “the AC magnetoconductivity at room temperature is 57% (af = 254 kHz) and 40% (af = 10 Hz) in a magnetic field B = 500 mT for the sample containing 40% by weight of magnetite nanoparticles.
This value is much higher than that obtained in DC (direct current) magnetotransport, where room temperature magnetoconductivities for similar compounds are typically 10% or less in comparable magnetic fields.
All of this has implications for the efficiency of 2D monolayer graphene transistors, whose electrons can operate at higher resistances”.
Final thoughts
Graphene spintronics shows that nanoscale circuits and transistors can be created that could be perfectly inoculated by a “vaccine”.
It also shows that magnetic electrodes are needed to obtain transistors and other spintronic devices.
This would once again explain the magnetic phenomenon of people inoculated with “vaccines”.
Along with this, it is also shown that ferromagnetic electrodes can be made from Fe3O4 magnetite, which coincides with typical combinations of graphene oxide and its many applications, e.g. aerosol injection into the atmosphere, aerogels, hydrogels, cancer therapeutic nanoparticles, gene therapies, drug delivery, biocides, fertilizers, where all the uses and related properties are explained in detail.
With all the information analyzed so far, it is hypothesized that the materials needed for the assembly of spintronic devices (i.e., graphene oxide and Fe3o4, or other materials not yet identified) are found in “vaccines” and are also indirectly introduced into the human body through food, water, air, and physiological serums (and probably in many other ways).
However, it remains to be clarified whether there are nanotechnologies or nanobots capable of assembling said material to achieve its operational capability.
Nevertheless, this does not exclude the possibility that materials can self-assemble through electromagnetic fields, by means of “Teslaphoresis” (Bornhoeft, LR; Castillo, AC; Smalley, PR; Kittrell, C.; James, DK; Brinson, BE; Cherukuri, P. 2016), a magnetic movement aimed at the self-assembly of carbon nanotubes, for which it requires a Tesla coil that generates an electromagnetic field or a potential difference between electrodes, as shown for example in this video.
Of course, Tesla’s coil could be replaced by the electromagnetic waves emitted by 5G antennas.
If the presence of magnetite Fe3O4, or some other ferromagnetic material, or some other nanometer electrode mechanism could be demonstrated, this theory could seem to be verified, since no specific order of nanomaterials would be required, as they would be found in the aqueous solution of the “vaccine”.
Assuming that the availability of spintronic devices is very likely, it is worth considering the capabilities and possibilities of this technology.
It seems logical that transistors and circuits would have the function of identifying the device, this would be the MAC (Media Access Control) address, which is a 48-bit unique identifier for its connection to the network.
Obviously, if there is a network device, there should be a mechanism for it to connect, or at least log on.
This requires a radio device responsible for modulating and transmitting the signal, and a controller on the other end in the form of a basic CPU with a digital signal processor and link controller.
Many electronic devices, usually connected via Bluetooth, meet these requirements, so it is conceivable that graphene transistors could be connected to the network in the same way as the IOT (Internet of Things).
In fact, according to (Yang, X., Liu, G., Balandin, AA and Mohanram, K. 2010), they have succeeded in creating “a single-transistor graphene amplifier that exploits the key concept of polarization in analog circuits…Compared to conventional amplifiers based on unipolar devices, the proposed single-transistor amplifier offers greater controllability in the field as it can switch between modes during operation.
To the best of our knowledge, this is the first work to demonstrate that a single transistor amplifier based on a three-terminal device can be configured in the field to operate as both a common source amplifier and a common well amplifier“.
And to all this, they add that graphene transistors can use modulation and coding techniques to establish communication or link with other devices, in fact “both PSK (phase shift keying) and FSK (frequency shift keying) are important digital modulation techniques.
PSK is widely used in wireless applications such as Bluetooth, Radio Frequency Identification (RFID), and Zig-Bee, while FSK is commonly used in radio and audio systems“.
Another relevant detail of this work (Yang, X., Liu, G., Balandin, AA and Mohanram, K. 2010) is the operating signal frequency of the developed transistors: 4, 8 and 10 KHz.
These data could be used for further research on graphene spintronic transistors.
Other work also supports the development of wireless graphene nano-transceivers (Iannazzo-Soteras, ME 2017) or graphene-based biorecognition transistors for biomedical applications connected via Bluetooth (Arora, K. 2019).
Bibliography
1.Akram, KB; ul-Hassan, SM; Ahmed, A.; Hamayun, MA; Rafique, M.; Manzoor, S. (2020). Giant AC magnetoconductivity in rGO-Fe3O4 composites. Journal of Magnetism and Magnetic Materials, 499, 166174. https://doi.org/10.1016/j.jmmm.2019.166174
2.Arora, K. (2019). Giant AC magnetoconductivity in rGO-Fe3O4 composites. Journal of Magnetism and Magnetic Materials, 6, 297.https://doi.org/10.1002/9781119468455.ch96
3.Avdoshenko, SM; Ioffe, IN; Cuniberti, G.; Dunsch, L.; Popov, AA (2011). Organometallic Complexes of Graphene: Toward Atomic Spintronics Using a Graphene Web. ACS nano. Handbook of Graphene, 5 (12), pp. 9939-9949. https://doi.org/10.1021/nn203719a
4.Bornhoeft, LR; Castillo, CA; Smalley, PR; Kittrell, C.; James, DK; Brinson, BE; Cherukuri, P. (2016). Teslaphoresis of carbon nanotubes. ACS nano, 10 (4), pp. 4873-4881.https://doi.org/10.1021/acsnano.6b02313
5.Braun, M.; König, J.; Martinek, J. (2006). RFrequency-dependent current noise through quantum-dot spin valves. Physical Review B, 74 (7), 075328. https://doi.org/10.1103/PhysRevB.74.075328
6.Bu, Y.; Liang, H.; Gao, K.; Zhang, B.; Zhang, X.; Shen, X.; Zhang, J. (2020). Wafer-scale fabrication of high-purity reduced graphene oxide films as ultrahigh-frequency capacitors with minimal self-discharge. Chemical Engineering Journal, 390, 124560. https://doi.org/10.1016/j.cej.2020.124560
7.Burkard, G.; Engel, HA; Loss, D. (2000). Spintronics and quantum dots for quantum computing and quantum communication. Fortschritte der Physik : Progress of Physics, 48 (9-11), pp. 965-986. https://doi.org/10.1002/1521-3978(200009)48:9/11%3C965::AID-PROP965%3E3.0.CO;2-V
8.González-Herrero, H.; Gómez-Rodríguez, JM; Mallet, P.; Moaied, M.; Palacios, JJ; Salgado, C.; Brihuega, I. (2016). Atomic-scale control of graphene magnetism by using hydrogen atoms. Science, 352 (6284), pp. 437-441. https://doi.org/10.1126/science.aad8038
9.Iannazzo-Soteras, ME (2017). Design exploration and measurement benchmark of integrated-circuits based on graphene field-effect-transistors : towards wireless nanotransceivers. http://hdl.handle.net/2117/113288
10.Jasim, DA; Lozano, N.; Kostarelos, K. (2016). Synthesis of few-layered, high-purity graphene oxide sheets from different graphite sources for biology. 2D Materials, 3 (1), 014006. https://doi.org/10.1088/2053-1583/3/1/014006
11.Jozsa, C.; van Wees, BJ (2019). Graphene Spintronics. En Spintronics Handbook : Spin Transport and Magnetism, Second Edition. pp. 121-154. https://doi.org/10.1201/9780429441189
12.Kharissova, OV; Garcia, BO; Kharisov, BI; Mendez, UO (2016). Magnetic-Graphene-Based Nanocomposites and Respective Applications. In Advances in Carbon Nanostructures. IntechOpen. http://dx.doi.org/10.5772/64319
13.Konwar, S.; Dhapola, PS; Gupta, M.; Singh, RC; Singh, PK (2019). High purity graphene oxide using electrochemical synthesis and its application. En Macromolecular Symposia (Vol. 388, No.1, p. 1900038). https://doi.org/10.1002/masy.201900038
14.Leuenberger, Minnesota; Perdita, D. (2001). Spintronics and quantum computing : switching mechanisms for qubits. Physica E : Low-dimensional Systems and Nanostructures, 10 (1-3), pp. 452-457. https://doi.org/10.1016/S1386-9477(01)00136-9
15.Li, L.; Qin, R.; Li, H.; Yu, L.; Liu, Q.; Luo, G.; Lu, J. (2011). Functionalized Graphene for High-Performance Two-Dimensional Spintronics Devices. ACS nano, 5 (4), pp. 2601-2610. https://doi.org/10.1021/nn102492g
16.Liao, ZM; Wu, HC; Wang, JJ; Cross, GL; Kumar, S.; Shvets, IV; Duesberg, GS (2011). Magnetoresistance of Fe3O4-graphene-Fe3O4 junctions. Applied Physics Letters, 98 (5), 052511. https://doi.org/10.1063/1.3552679
17.Gerg, NM ; Bender, SA; Duine, RA; Schuricht, D. (2018). Spin switching via quantum dot spin valves. Physical review letters, 120 (1), 017701. https://doi.org/10.1103/PhysRevLett.120.017701
18.Hashmi, A.; Hong, J. (2014). Magnetic properties of graphene/BN/Co(111) and potential spintronics. Journal of magnetism and magnetic materials, 355, pp. 7-11. https://doi.org/10.1016/j.jmmm.2013.11.036
19.Maassen, J.; Ji, W.; Guo, H. (2011). Graphene spintronics: the role of ferromagnetic electrodes. Nano letters, 11 (1), pp. 151-155. https://doi.org/10.1021/nl1031919
20.Manoratne, CH; Rosa, SRD; Kottegoda, IRM (2017). XRD-HTA, UV Visible, FTIR and SEM Interpretation of Reduced Graphene Oxide Synthesized from High Purity Vein Graphite. Material Science Research India, 14 (1), pp. 19-30. http://dx.doi.org/10.13005/msri/140104
21.Ray, SC; Soin, N.; Makgato, T.; Chuang, CH; Pong, WF; Roy, SS; McLaughlin, JA (2014). Graphene supported graphene/graphene bilayer nanostructure material for spintronics. Scientific Reports, 4 (1), pp. 1-7. https://doi.org/10.1038/srep03862
22.Rosillo-Lopez, M.; Salzmann, CG (2016). A simple and mild chemical oxidation route to high-purity nano-graphene oxide. Carbon, 106, pp. 56-63. https://doi.org/10.1016/j.carbon.2016.05.022
23.Soriano, D.; Munoz-Rojas, F.; Fernández-Rossier, J.; Palacios, JJ (2010). Hydrogenated graphene nanoribbons for spintronics. Physical Review B, 81 (16), 165409. https://doi.org/10.1103/PhysRevB.81.165409
24.Tsuchiya, T.; Terabe, K.; Ochi, M.; Higuchi, T.; Osada, M.; Yamashita, Y.; Aono, M. (2016). In situ tuning of magnetization and magnetoresistance in Fe3O4 thin film achieved with all-solid-state redox device. Acs Nano, 10 (1), pp. 1655-1661. https://doi.org/10.1021/acsnano.5b07374
25.Wang, J.; Xu, X.; Mu, X.; Ma, F.; Sun, M. (2017). Magnetics and spintronics on two-dimensional composite materials of graphene/hexagonal boron nitride. Materials Today Physics, 3, pp. 93-117. https://doi.org/10.1016/j.mtphys.2017.10.00327
26.Garrese, F.; Dubois, M.; Savchenko, AK (2010). Electron properties of fluorinated single-layer graphene transistors. Physical review B, 82 (7), 073403. https://doi.org/10.1103/PhysRevB.82.073403
27.Yang, X., Liu, G., Balandin, AA e Mohanram, K. (2010). Triple-Mode Single-Transistor Graphene Amplifier and Its Applications. ACS nano, 4 (10), pp. 5532-5538. https://doi.org/10.1021/nn1021583
28.Zhao, MQ; Zhang, Q.; Huang, JQ; Tian, GL; Chen, TC; Qian, WZ; Wei, F. (2013). Towards high purity graphene/single-walled carbon nanotube hybrids with improved electrochemical capacitive performance. Carbon, 54, pp. 403-411. https://doi.org/10.1016/j.carbon.2012.11.055